
What happens in a Genetics laboratory? (Part II)
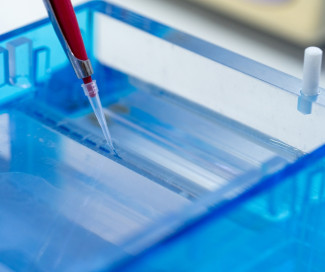
When there is suspicion of a genetic disease caused by a mutation in a specific gene, the physician will request a diagnostic test that involves analysing that particular gene in a molecular genetics laboratory. As we recall, the genetic instructions encoded within DNA are represented by four nucleotide letters: A (adenine), C (cytosine), G (guanine), and T (thymine). In the molecular genetics laboratory, scientists will detect any "spelling errors" or mutations within the sequence of the suspected gene.
A gene can consist of a sequence comprising 10,000 or more DNA letters of code. The dedicated task of a geneticist is to decipher this intricate code and identify potential alterations that could disrupt the organism's vital instructions, thus leading to the development of the disease.
Unlike chromosomes, which can be visualised under a microscope, DNA cannot be directly observed in the same manner. The molecular geneticist extracts the DNA from the patient's cells and employs a series of sophisticated chemical reactions to facilitate the decoding of the target gene's sequence. While numerous techniques exist for identifying mutations, sequencing remains one of the most prevalent and effective methods in use today.
Genetic sequencing
Sequencing is the fundamental process employed to determine the precise order of nucleotides (A, T, G, C) within a nucleic acid molecule, be it DNA (deoxyribonucleic acid) or RNA (ribonucleic acid). Over the past few decades, significant advancements have been made in sequencing methodologies and technologies, enabling rapid and accurate determination of nucleotide sequences.
Among the pioneering sequencing techniques developed, the Sanger method holds a prominent place. This method relies on chain termination DNA synthesis, where DNA fragments of varying lengths are generated and subsequently separated and read to unveil the complete sequence. At the core of Sanger sequencing lies the functionality of DNA polymerases, enzymes responsible for replicating DNA by creating a complementary strand based on a given template. Despite its labour-intensive nature, the introduction of the Sanger sequencing method in the late 1970s was revolutionary, and it played a pivotal role in sequencing the human genome through the Human Genome Project.
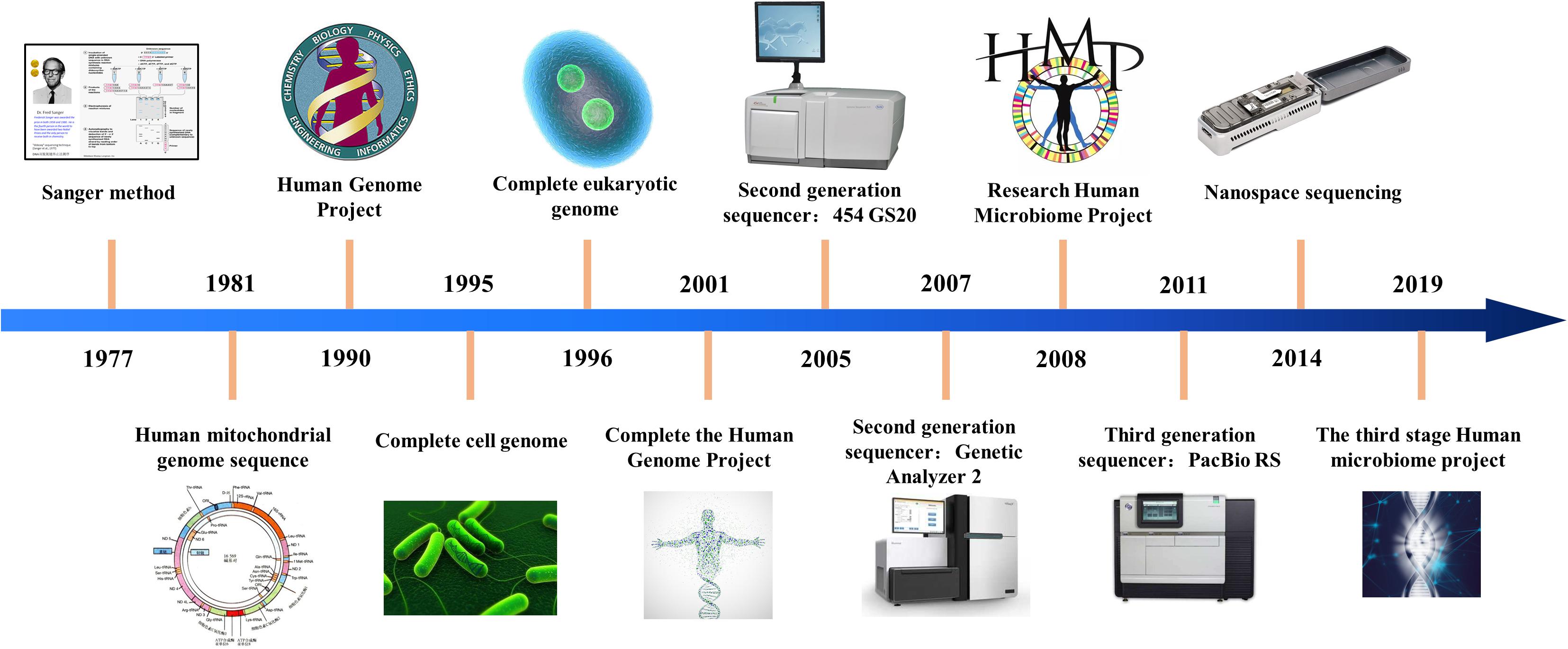
In recent years, the field of sequencing has witnessed significant advancements with the introduction of Next Generation Sequencing (NGS) technologies. These cutting-edge techniques encompass synthesis-based sequencing, nanopore sequencing, and single-molecule sequencing. One of their remarkable features is their ability to rapidly and cost-effectively sequence vast amounts of DNA.
The impact of sequencing on biomedicine has been profound. Beyond its crucial role in diagnosing genetic diseases, sequencing has revolutionised diverse areas of research. It plays a key role in studying species evolution and unraveling the intricacies of genetic diversity. In the field of oncology, sequencing has opened new avenues for research and personalised treatments. Furthermore, it has greatly contributed to the development of gene therapies, offering promising solutions for various genetic disorders.